How do quantum bits use magnets to "communicate" in novel designs?
Now, researchers at the University of Chicago, the U.S. Department of Energy's Argonne National Laboratory, the University of Iowa, and Tohoku University in Japan have begun to develop a device similar to the one described above, which utilizes the principle of connecting individual quantum bits on a chip via magnets, as opposed to transmitting signals through the air.
A related paper was published in the Proceedings of the National Academy of Sciences.
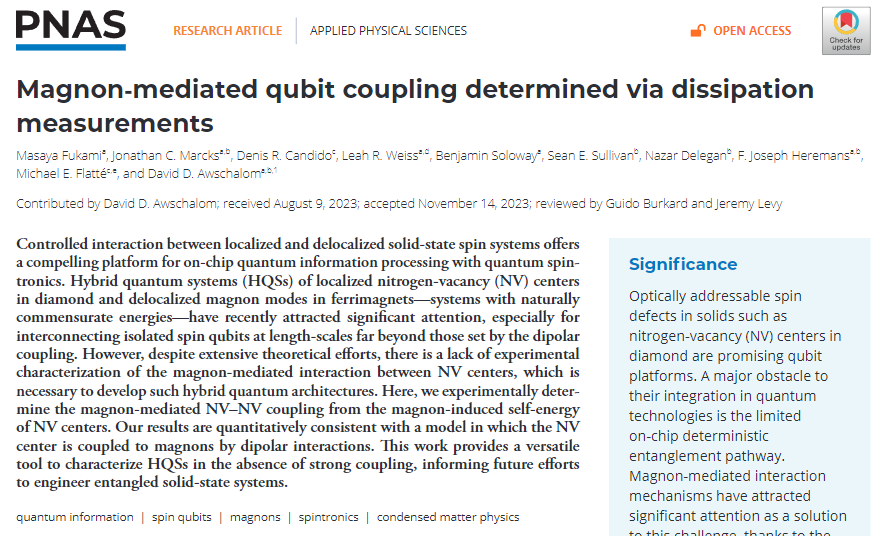

David Awschalom, the Liao Family Professor of Molecular Engineering and Physics at the University of Chicago's Pritzker School of Molecular Engineering, director of the Chicago Quantum Exchange, director of Q-NEXT, the Department of Energy's Center for Quantum Information Science chaired by Argonne, and the project's principal investigator. "This is a proof-of-concept for scalable, robust quantum technology using conventional materials at room temperature," explained Awschalom.
"The beauty of this experiment is its simplicity and the use of proven techniques to design and ultimately entangle quantum devices."
Connecting quantum bits via quantum entanglement is necessary to build quantum computers, but it's often tricky. Nitrogen vacancy (NV) centers can be used as defects in quantum bits in diamond, but the challenge is that in order to "talk" to each other, they must be very, very close together.
The maximum extent of normal quantum interactions between NV centers is only a few nanometers, or one-thousandth the width of a hair.
Michael Flatté, a professor of physics and astronomy at the University of Iowa, says, "Scientists need to be able to grab the inside with their hands, connect the wires and make devices; but they're too close in nanometers."
That's where magnets come in.
Two years ago, Fratté and his collaborators published a theoretical paper proposing the use of magnetic materials to create quantum connections between NV color centers so that they could become entangled at greater distances from each other.
In this study, normal interactions between two NV (nitrogen-vacancy) color centers are involved. Specifically, microwaves play a key role in this proposed device. A magnet is designed to receive a microwave signal from one NV color core and then transmit this signal to the other NV color core via so-called "magnets".
In a magnet, the spins of all the electrons inside point in the same direction, just as the stalks of grain all point upward; the magnets are perturbed by the slight ripples created by these spins - just as the wind ripples through a field of grain.
In fact, magnons can travel much farther than nanometers, or even a thousand times farther than nanometers, to many micrometers.
Fratté goes on to say, "The micrometer scale is very interesting because it is the typical scale of many integrated electronic devices, such as the silicon transistors in computer chips."
"So if something of this size can be made, then it will be possible to get a reasonable amount of it on a chip."
Connecting NV color-centered quantum bits with magnets also allows for selective interaction: if two quantum bits in a quantum computer "talk" at slightly different frequencies, they can become entangled without interfering with or being affected by other quantum bits, even if there are other quantum bits between them. there are other quantum bits between them.
This ability is extremely important for the complex tasks that scientists want quantum computers to perform.
The experiment by Oshalom and his collaborators successfully demonstrated that NV color centers can "talk" to magnetic materials and transmit microwaves in the form of magnons. In addition, the experimental data matched almost exactly the predictions made in a theoretical paper two years ago.


Masaya Fukami, first author of the paper, said, "This work is a good synergy between experiment and theory." Fukami was a postdoctoral fellow at the University of Chicago's Pritzker School of Molecular Engineering during the experimental period and now works at the quantum computing company PsiQuantum.
He said, "I was impressed by how accurately the model predicted the experiment. This gives me confidence in the system."
Now that they've established that the NV color core can "talk" to the magnet, the next step is to place another NV color core on the other side to see if the magnet can mediate a quantum connection between the two.
"This is the first integration model that uses magnets." Fraté exclaimed, "I think this is a very powerful approach that could in principle be applied to other solid-state quantum bit systems as well."